Comparison of Lagrangian Super-droplet and Eulerian Double-moment Bin Microphysics Schemes in Stratocumulus and Cumulus Clouds
Authors
Kamal Kant Chandrakar — National Center for Atmospheric Research (NCAR) *
Hugh Clifton Morrison — University Corporation for Atmospheric Research
Wojciech Grabowski — National Center for Atmospheric Research (NCAR)
George Bryan — National Center for Atmospheric Research (NCAR)
Category
Warm low clouds, including aerosol interactions
Description
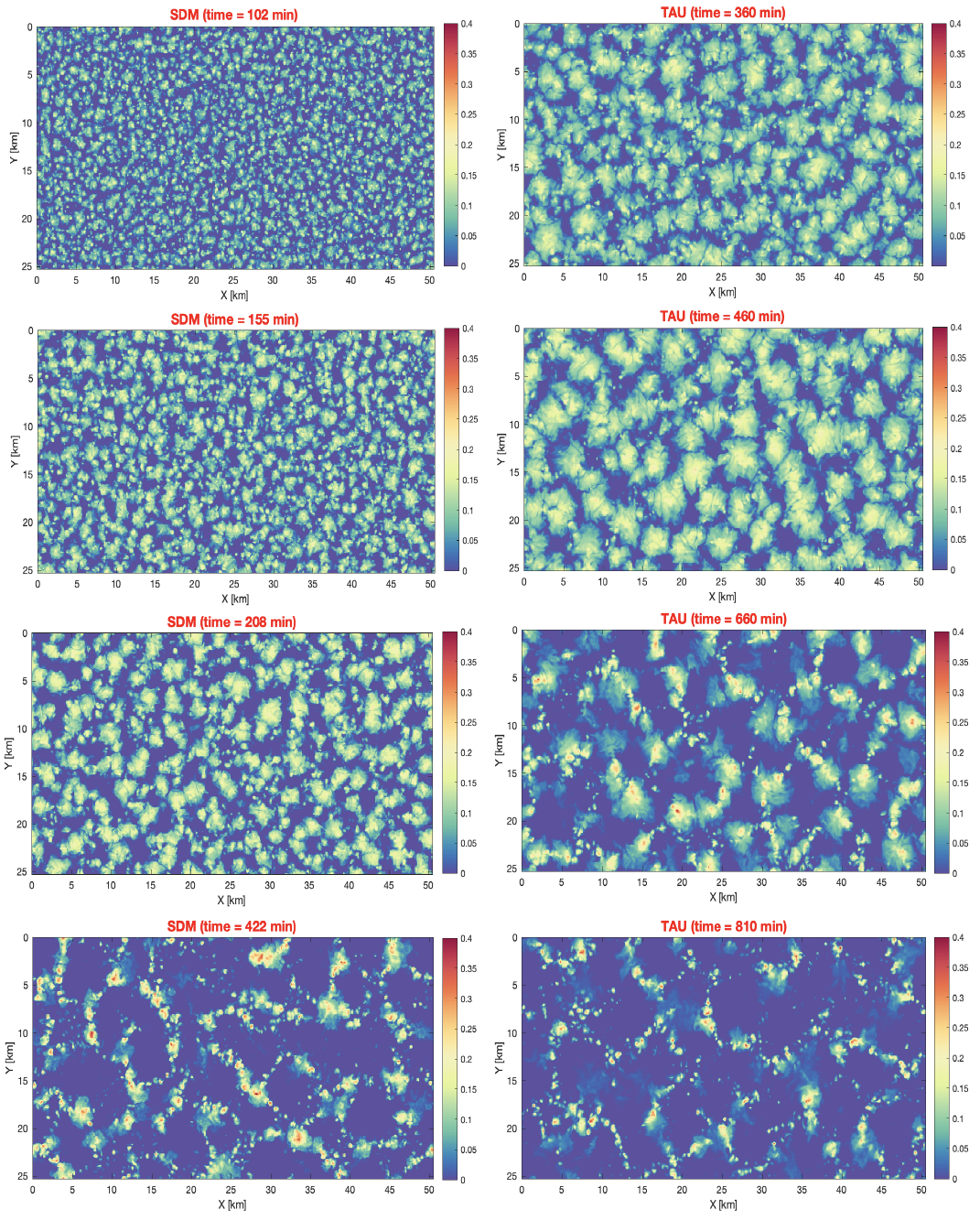
Advanced microphysics schemes (such as Eulerian bin and Lagrangian super-droplet) are becoming standard tools for cloud physics research and parameterization development. However, this leads to a question: how well do these schemes represent cloud processes? To answer this question, we compared a double-moment bin scheme and a Lagrangian super-droplet scheme via large-eddy simulations of stratocumulus and cumulus clouds. Two sophisticated microphysics schemes implemented in the CM1 model were used: (a) the super-droplet method (SDM) and (b) the Tel Aviv University (TAU) bin scheme. SDM tracks various properties of “super-droplets,” including drop size and solute mass, allowing representation of aerosol-cloud processing in a physically detailed way. In contrast, the bin approach of TAU (and most others) does not track the solute mass in drops. Thus, it cannot represent aerosol processing explicitly. Our implementation of TAU carries an additional twenty aerosol bins per grid box to represent activation, coalescence scavenging, and aerosol regeneration. However, during complete evaporation of droplets, their number is added back to the smallest unfilled aerosol bin relative to the initial distribution.
Limitations representing aerosol-cloud interactions in the bin scheme lead to differences in the evolution of the stratocumulus cloud field between TAU and SDM runs. The bin scheme significantly delays the transition of the stratocumulus cloud field from closed to open cells (see Fig. 1). We compared cloud properties between SDM and TAU simulations in closed and open cellular regimes. A relatively broader average droplet size distribution is evident in SDM runs. However, its vertical variation is nearly the same in TAU and SDM runs after the transition to open cells. Sensitivity tests with various treatments of aerosol scavenging and different initial aerosol conditions in TAU are analyzed to understand specific reasons for the differences in behavior than SDM. In contrast to the stratocumulus case, TAU simulations of an isolated cumulus cloud show a significantly larger standard deviation of cloud droplet radius than those using SDM, likely due to the numerical diffusion in TAU runs. For the precipitating cumulus case, larger spectral width in the TAU simulations initiates rain earlier and enhances precipitation development in a positive feedback loop. However, with time, rain formation in the SDM simulations begins to catch up to the TAU simulations.
Lead PI
Wojciech Grabowski — National Center for Atmospheric Research (NCAR)