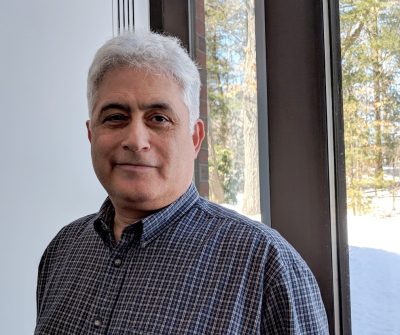
A onetime string theorist now improves the way models represent spectral radiances throughout the atmosphere
Physicist Eli Mlawer (rhymes with “flower”) is a principal scientist at Atmospheric and Environmental Research (AER), a for-profit research company in Lexington, Massachusetts, where he develops algorithms that help modelers represent radiative transfer.
Several times since 2008, Mlawer’s work has been funded by the Atmospheric System Research (ASR) program of the U.S. Department of Energy (DOE), with an aim to improve the treatment of atmospheric radiation in DOE-supported regional and global earth system models.
Atmospheric radiative transfer determines how much energy in the form of electromagnetic radiation is either absorbed by the Earth or scattered back into space. Radiative heating is the engine that drives the Earth’s atmospheric and hydrological circulation.
Evaluating and improving parameterizations—mathematical representations—of atmospheric radiative processes requires a high volume of high-quality spectrally resolved radiation measurements from diverse climate regions around the globe.
For most of such data, Mlawer credits DOE’s aptly named Atmospheric Radiation Measurement (ARM) user facility, which maintains both fixed and portable atmospheric observatories in climate-critical regions across the world.
Improving radiative transfer calculations was a primary critical objective within ARM, which started in 1992. Mlawer explained those early objectives as co-author of two chapters in a book-length monograph on ARM history and contributions published in 2016 by the American Meteorological Society.
To this day, says Mlawer, ARM provides “a sound observational base for what radiative calculations should be.”
Making Calculations Faster
When Mlawer arrived at AER in 1994, spectral radiance calculations for earth system models were provided by the AER-developed Line-By-Line Radiative Transfer Model (LBLRTM). It was a product of AER developers, including early ARM data user and LBLRTM pioneer S.A. “Tony” Clough.
An updated version—the most recent of many—is available on the company’s menu of free software for modelers.
“The (atmospheric science) community had gained confidence in the calculations of LBLRTM,” he says. “It was accurate, but it was far too slow to run in a climate model.”
AER wanted to make a new model that was fast, accurate, and consistent with the newest observations, including those at ARM atmospheric observatories.
Just months after finishing a physics dissertation on string theory at Brandeis University in Waltham, Massachusetts (PhD, 1994), Mlawer took the lead in designing, implementing, and validating a rapid radiative transfer model (RRTM) that was orders of magnitude faster than LBLRTM alone.
What took “millions and millions of calculations to do” with LBLRTM, says Mlawer, “RRTM boiled down to about 200.”
However, RRTM and its second-generation spinoffs (RRTMG and RRTMGP) are still more computationally expensive to run than Mlawer and his collaborators would like.
“We’re working on that,” he says.
“We” refers to a long-term collaboration he has with Robert Pincus, a research scientist with the Cooperative Institute for Research in Environmental Sciences in Colorado.
Into the Infrared
Mlawer’s latest ASR project as a principal investigator (PI)—on climate-relevant gas absorption properties from ARM spectral measurements—shares a research thread with his others: to investigate the spectroscopy that underlies data on radiative transfer and to improve knowledge of atmospheric radiative processes.
Infrared spectroscopic parameters, including the water vapor continuum, are important to radiative balance, remote sensing, and data assimilation. (At AER, Mlawer is lead developer of the MT_CKD water vapor continuum model.)
“Understanding the spectroscopic properties of water vapor, a greenhouse gas, will give you a better handle on how radiative transfer works in the atmosphere,” he says.
One puzzle of the atmosphere’s strong water-vapor absorption bands in the mid-to-upper troposphere led Mlawer and co-PI David Turner, a National Oceanic and Atmospheric Administration (NOAA) meteorologist, to design and execute two ARM field campaigns. They aimed to explore the largely unexplored far-infrared (far-IR) part of the electromagnetic spectrum.
The Critical Far-IR
About 40 percent of the longwave (thermal) radiation leaving Earth is emitted by water vapor transitions in the far-IR band. The same part of the spectrum also provides most of the longwave cooling in the upper troposphere. Yet because of the opacity of the lower atmosphere, this climate-critical band in the mid-to-upper troposphere is hard to measure with ground-based instruments.
Mlawer and Turner decided to leverage the quality of a new generation of far-IR instruments by taking sustained clear-sky measurements in two of the world’s driest places. Extremely low water vapor loadings in such locales greatly reduce the lower-atmosphere opacity that impedes studies of the far-IR.
In 2007, their Radiative Heating in Underexplored Bands Campaign (RHUBC, pronounced “roobik”) took place at ARM’s North Slope of Alaska atmospheric observatory. In 2009, they followed with RHUBC-II at an arid high-altitude site in the Atacama Desert of mountainous northern Chile.
“Both were demanding,” says Mlawer, who co-authored a dual campaign summary with Turner. In Alaska, it was the middle of winter and 40 degrees below zero. In Chile, for a month, each scientist visited the frigid campaign site for up to five hours every other day, at an altitude of 17,650 feet (5,380 meters)—nearly 3 1/2 miles up.
A paper summarizing what they learned from RHUBC-II will be published soon, outlining improved far-IR spectroscopic parameters for models.
Despite hardships, their two arid-clime campaigns improved water vapor spectroscopy, says Mlawer. “And they were highlights of my career.”
Good Fortune
Mlawer, a native of North Bellmore, New York, had an early passion for numbers. He applied that to baseball statistics in his youth, Math Olympics in high school, and to an academic career that included three degrees worth of study in mathematics, astrophysics, physics, and (for his physics doctorate) string theory.
At Williams College in Williamstown, Massachusetts (B.A., astrophysics and mathematics, 1982), Mlawer kept a primary focus on physics. It took a while, however, for physics to lead him to investigating atmospheric radiation.
For two years starting in 1982, he was a Herschel Smith postgraduate fellow at Emmanuel College of the University of Cambridge in England (B.A., physics, 1984). After that idyll of intellectual excitement and wide travel, Mlawer worked for a Boston-area software company while his wife started a career in business.
The two met in elementary school on Long Island, New York, dated through high school and college, married after the Cambridge sojourn, and now have three adult children.
His doctoral studies at Brandeis were in the early days of string theory, a path of study that touches on questions of theoretical physics and attempts to explain a lot of physics, including quantum gravity. It eventually became the focus of his dissertation.
Intellectually, in string theory, the sky was the limit—the physics of black holes and condensed matter, the cosmology of the early universe—but the real sky was nowhere in sight.
“I didn’t even mention the word ‘atmosphere’ in my graduate school years,” he says.
After earning his PhD, however, Mlawer was determined to go in a different direction than string theory, “which is notorious for being unverifiable,” he says. “I knew I would be looking for jobs that had a connection to what was going on in the world.”
Part of that practical urge came from his sense of family. Mlawer is a first-generation American whose Ukrainian-born mother, a retired social worker, was a refugee following World War II after surviving the Holocaust.
His father, a onetime business manager at the Simon & Schuster publishing company, was born in Cuba to immigrants who fled Eastern Europe after World War I. (The family name derives from “Mlawa,” his paternal grandfather’s birthplace in north-central Poland.)
Family history “informed quite a bit of my perspective,” he says. “I realized that I needed and wanted to work on problems that are directly relevant to mankind.”
At AER, Mlawer embarked on a long career devoted in part to nudging earth system models closer to the realities of atmospheric radiative energy.
The research freedom and excitement are equivalent to being at a university, says Mlawer, and getting to AER was “good fortune.”
# # #
This work was supported by the U.S. Department of Energy’s Office of Science, Office of Biological and Environmental Research as part of the Atmospheric System Research Program.