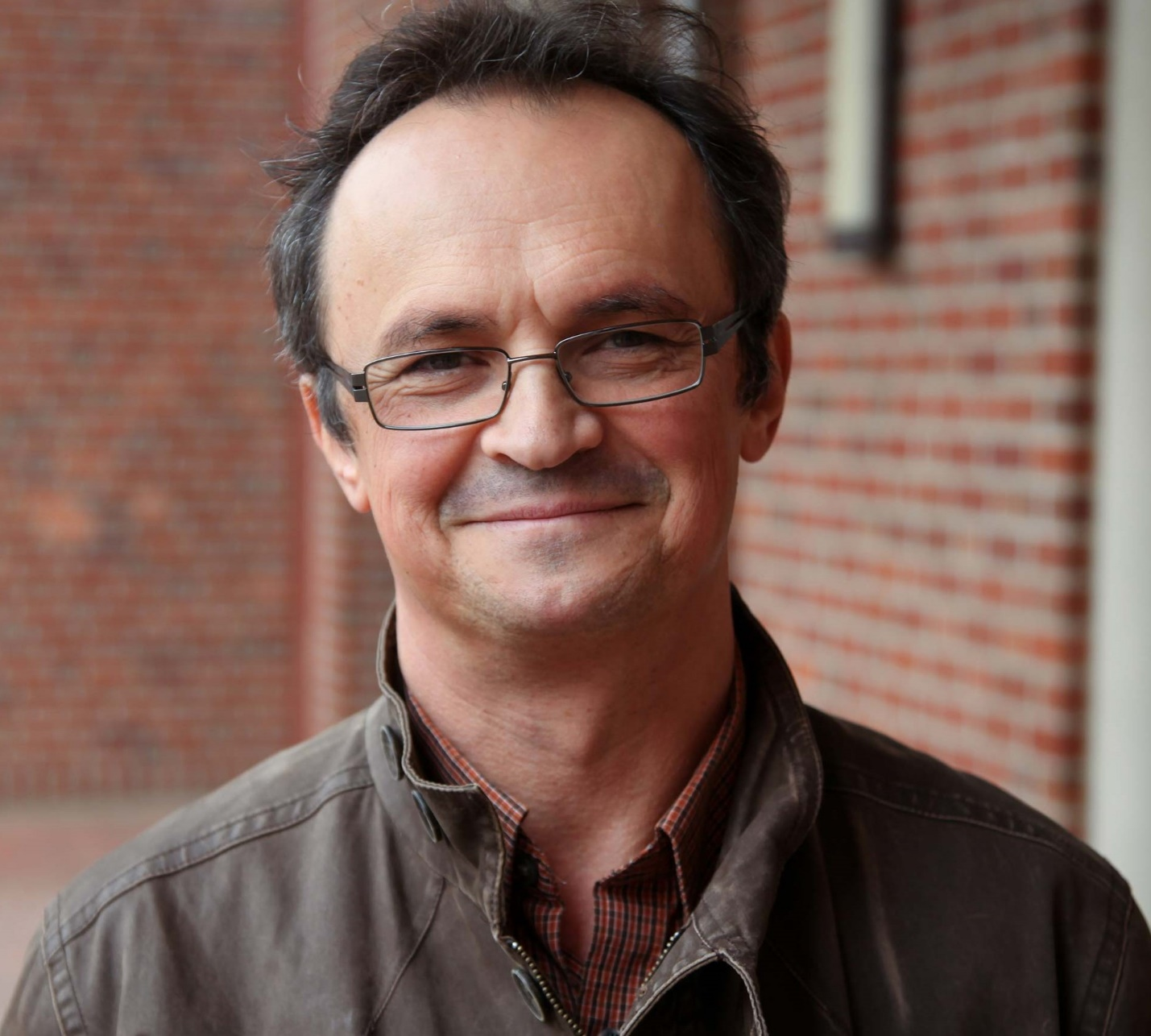
One scientist puzzles out the dynamism of atmospheric aerosols and their ever-changing chemical transformations
From his teaching and research post at Purdue University in Indiana, analytical and physical chemist Alexander Laskin belongs to a worldwide cohort of scientists investigating particles in the atmosphere called aerosols.
Each as different as snowflakes and countable (if at all) in the quadrillions, aerosols are suspensions of solid and liquid particles above the surface of Earth.
Profoundly consequential to weather and climate, aerosols absorb and scatter radiation from the sun and the Earth. They also determine how clouds form, their physical properties, and how long they last.
Aerosols are dynamic, highly reactive chemically, and change shape as they age. They have high surface areas and a lot of water content, which means their chemistry is multiphase―a state of being so mutable that the composition and properties of aerosols change constantly.
Imaging Studies
Laskin grapples with the gap between the chemical details of such particles and the practical needs of the models that simulate atmospheric processes.
At Purdue, his research group focuses on developing and applying new ways to image and analyze the chemistry of aerosols, down to the level of molecules and individual particles. Broadly, their goal is to investigate the effect of aerosols on atmospheric chemistry, air pollution, and the climate.
At the molecular level, the group’s imaging techniques and studies involve travel to the Environmental Molecular Sciences Laboratory (EMSL), a U.S. Department of Energy (DOE) user facility. EMSL is located on the campus of Pacific Northwest National Laboratory (PNNL) in Richland, Washington, where Laskin was a researcher from 1999 to 2017.
Today, Laskin and his group at Purdue are fully engaged in a 2018–2021 aerosols chemistry project for DOE’s Atmospheric System Research (ASR) program.
They use spectromicroscopy to describe the physical and chemical properties of atmospheric particles collected during field campaigns by DOE’s Atmospheric Radiation Measurement (ARM) user facility. ARM maintains fixed and portable observatories in the world’s climate-critical regions and for more than two decades has supported fieldwork on every continent.
With spectromicroscopy, it’s possible to get X-ray absorption images that yield data on characterized elements and chemical species from artifacts as small as a 20-nanometer pixel.
Tiny and Changeable
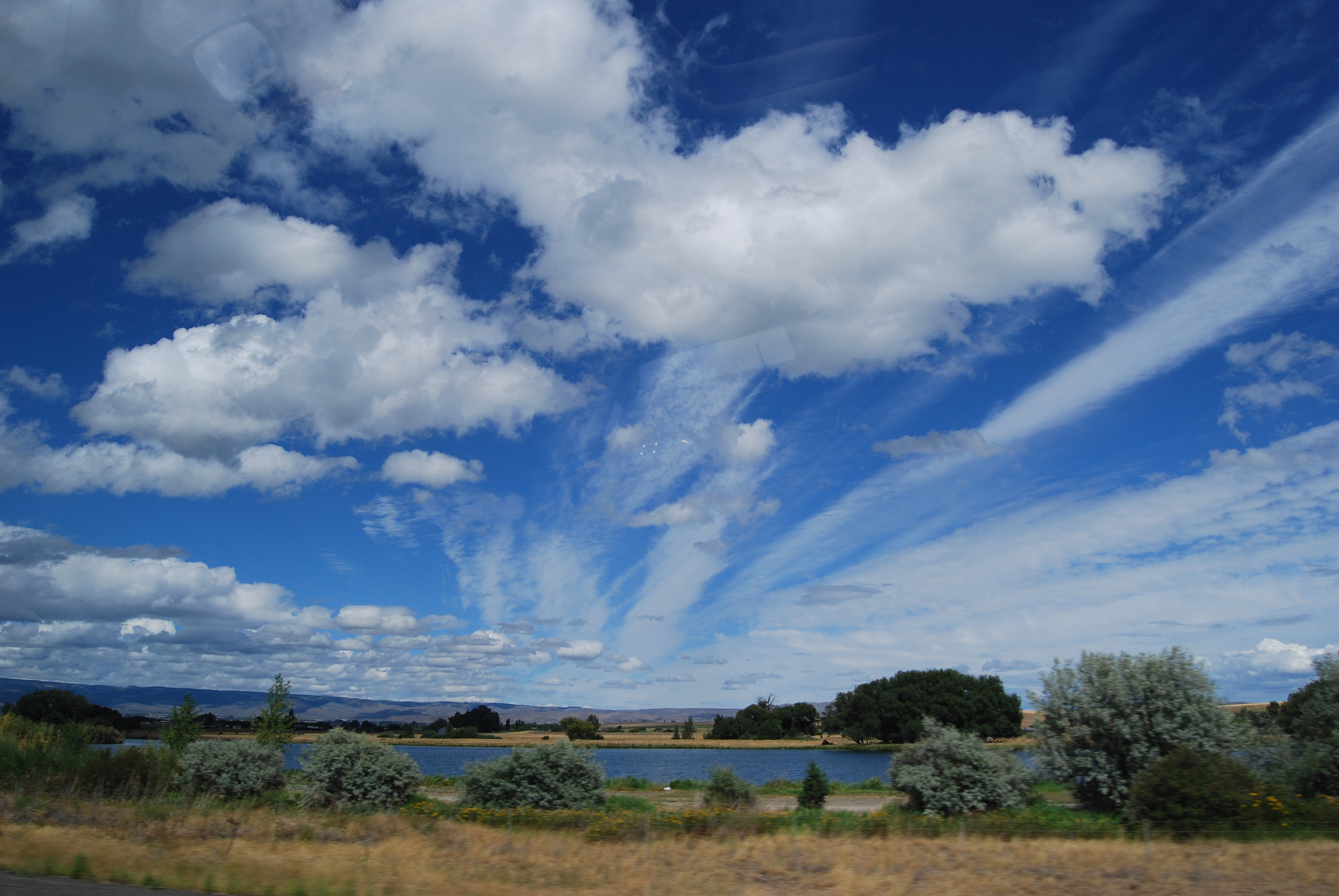
The defining characteristic of aerosols is negligible terminal fall speeds: that is, they are too light to fall and therefore do all their important work while suspended in the atmosphere.
The primary particles that contribute to aerosols come from the surface of the Earth as mineral-rich dust and sea spray; from power plants and transportation as soot and organic particles emitted by combustion engines; and from fragments of plants and animals. Others, called “secondary” particles, are formed in the atmosphere itself.
To add spice, aerosols cover a wide range of sizes. They can be as small across as a few nanometers or tens of micrometers―huge, in atmospheric terms.
Laskin asks: “How can we take this complexity and use it in some practical way?”
He and others know that aerosols are important to the formation of warm clouds and ice crystals within cold clouds. But these small specks also prompt big questions.
“We know there are no two particles alike―that they are multicompetent systems,” says Laskin. “But the question is, how do we find out the variability of the internal composition of these particles? And how can the enormous complexity of particles be packed together―parameterized―for practical use in climate models?”
Particle-Picture Challenges
Getting a full chemical and physical picture of single atmospheric particles at nanometer scales is challenging because of particle complexity, including the shapes and internal distribution of their various components.
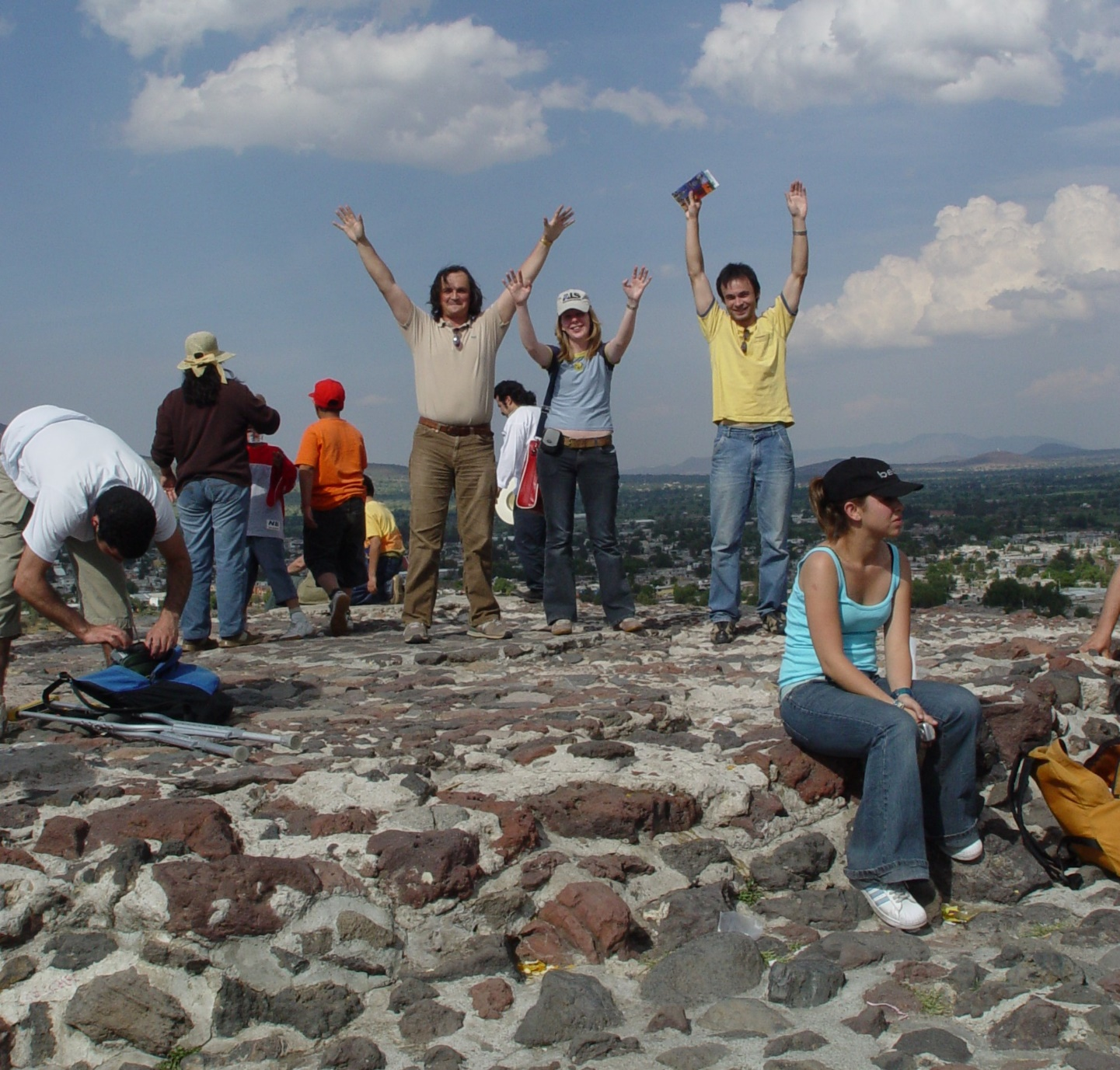
Laskin has been in this research world since the late 1990s and outlined chemical imaging advances in a 2019 review article.
His two coauthors were air-quality analyst Ryan Moffet of Sonoma Technology, Inc. in California and Mary K. Gilles of Lawrence Berkeley National Laboratory (LBNL), who recently retired. The three have been working together for more than 15 years.
LBNL is home to Advanced Light Source, a DOE user facility with a synchrotron, to which members of the Laskin group have also frequently traveled. Such machines, the size of a football field, accelerate electrons to create extremely bright light by sending them through magnetic fields.
“One of the synchrotron-based instruments we heavily use is an X-ray microscope, which requires a unique source of light,” says Laskin of leaning on LBNL. “It’s a technology that can only be done in these unique facilities.”
Data from Campaigns
For the ASR project, Laskin and his research team get observational data primarily from ARM’s 2017–2018 Aerosol and Cloud Experiments in the Eastern North Atlantic (ACE-ENA) field campaign.
They are also tapping measurements from other field campaigns: the Aerosol-Ice Formation Closure Pilot Study, conducted at ARM’s Southern Great Plains (SGP) atmospheric observatory; Holistic Interactions of Shallow Clouds, Aerosols, and Land-Ecosystems (HI-SCALE), which took place in 2016 at the SGP; and Observations and Modeling of the Green Ocean Amazon (GoAmazon2014/15), which spanned 2014 and 2015 in Brazil.
In the latter three field campaigns, aerosols from plants and soil played a big part. While he was at PNNL, Laskin was senior author on a 2016 Nature Geoscience paper demonstrating that as much as 60 percent of airborne particles after a rainstorm were from soil organics bounced into the air by raindrops.
Similarly surprising results were recorded in a 2018 Nature Communications paper with Laskin as a senior author. It dispelled the notion that atmospheric particles containing sodium were always from marine sources like sea spray. GoAmazon2014/15 data showed that similarly salty particles, in abundance, originate from tropical forests by way of fungal spores.
A Wide Net of Collaborators
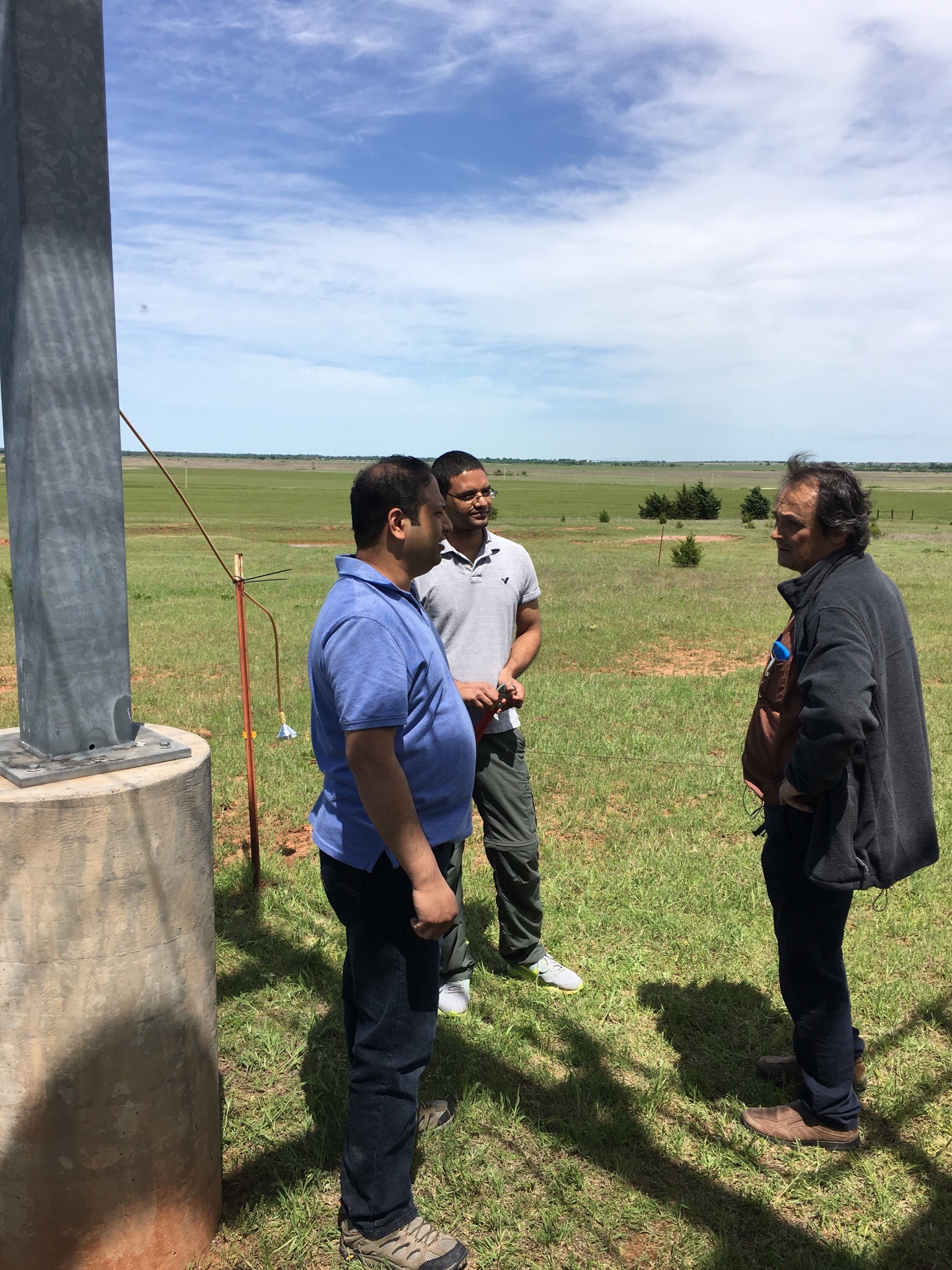
Laskin’s ASR project depends on collaborators for unique insights into the chemistry of atmospheric particles.
At Purdue, his colleagues focus on single-particle views of ambient atmospheric particles to tease out their chemical composition.
At EMSL, PNNL atmospheric chemist Swarup China leads collaborative research on particle characterization.
PNNL chemist Alla Zelenyuk-Imre “is the single-particle mass spectrometry expert,” says Laskin. “She developed unique instruments for probing individual particles in real time.”
Daniel Knopf of Stony Brook University in New York focuses on the propensity of atmospheric particles to nucleate ice. He led the 2019 Aerosol-Ice Formation Closure Pilot Study at the SGP.
Jian Wang of Washington University in St. Louis, who was the lead scientist for ACE-ENA, studies aerosols and cloud formation in the mid-Atlantic Ocean.
Other collaborators, PNNL atmospheric scientists Jerome Fast and Larry Berg, led HI-SCALE.
“We work side by side,” says Laskin of his collaborators, a mix of experts in fundamental chemical analysis and the fundamental processes of cloud formation. “It’s chemistry and microphysics, stitched together. The data we create is very complementary.”
Growing up Scientific
Laskin grew up in St. Petersburg, in the former Soviet Union. He was the son of parents who were both chemical engineers.
“Science and research were somehow cultivated in the family,” he says, “and I always did well in science classes, though I did not have a specific focus.”
When he enrolled at St. Petersburg Polytechnic University (M.S., 1990). it was to study mechanical engineering. He met his wife there, a fellow undergraduate.
Julia Laskin, an analytical chemist who worked at PNNL as a mass spectrometry scientist and was a lab fellow, now teaches and directs a research team at Purdue.
“Each of us have our own research areas, but we overlap,” says Laskin.
Upon graduating, they both emigrated to Israel―married by then and the parents of a 1-year-old son. (Today, their son, Michael, is 29; daughters Olga and Shani are 22 and 17, respectively.)
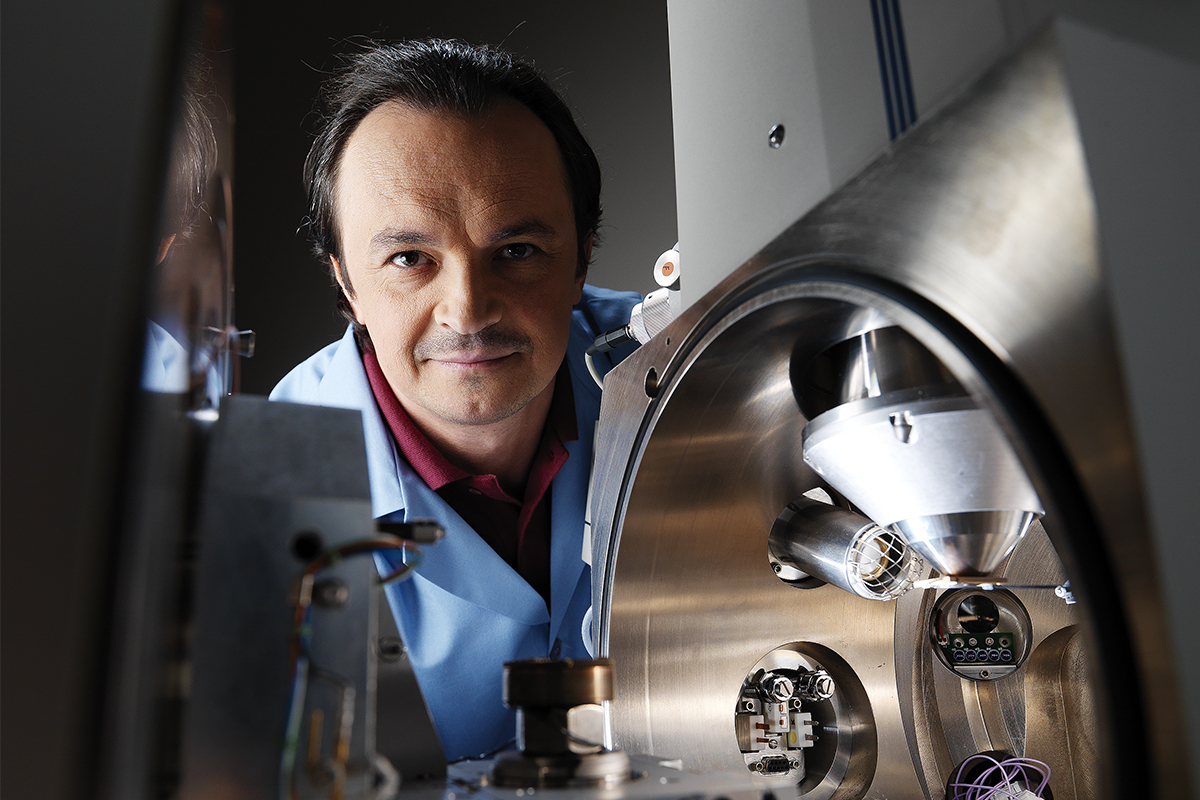
“It was the last year of the Soviet Union,” says Laskin. “The Iron Curtain was removed and we decided not to stay in that system any longer.”
Along with his wife, he pursued doctoral work in physical chemistry at the Hebrew University of Jerusalem (PhD, 1998).
“Professionally, I was shaped there. I did no deep research until Jerusalem,” says Laskin, describing the years he and Julia were mentored by another professional couple, Assa and Chava Lifshitz. “We lived this science-family life.”
Along the way, he attended conferences in western Europe and embraced the vibrant culture of urban Israel. That meant moving to the United States was not a shock at all, says Laskin, “other than the very long flights.”
‘An Absolutely Unique Environment’
Starting in 1998, the couple spent a year as postdoctoral researchers at the University of Delaware, where mass spectrometry pioneer Jean Futrell was Julia’s advisor.
In 1999, Futrell was named to direct EMSL and invited the couple to follow him. (EMSL had opened only two years earlier.)
At PNNL, though still a postdoc, at first, and primarily a gas-phase chemist, Laskin was challenged to create a user group to investigate the chemistry of atmospheric aerosols.
“It was a very quick way to get integrated into the (PNNL) community,” says Laskin, and to challenge external users to take part.
EMSL was just right for that, he adds―“an absolutely unique environment, with a broad range of technologies.”
At the time, DOE and the programmatic precursors of ASR were eager to bring EMSL into jump-starting research on aerosol chemistry and its effect on climate. That included more research on the microscopic and molecular information on atmospheric particles.
Laskin’s work with Moffet and Gilles started in earnest around 2005. All three were supported by funding from ASR and the DOE programs that came before it.
Today, he and others are still trying to close knowledge gaps regarding the multiphase chemistry of atmospheric aerosols.
“It is really a very complex puzzle,” says Laskin.
# # #This work was supported by the U.S. Department of Energy’s Office of Science, through the Biological and Environmental Research program as part of the Atmospheric System Research program.