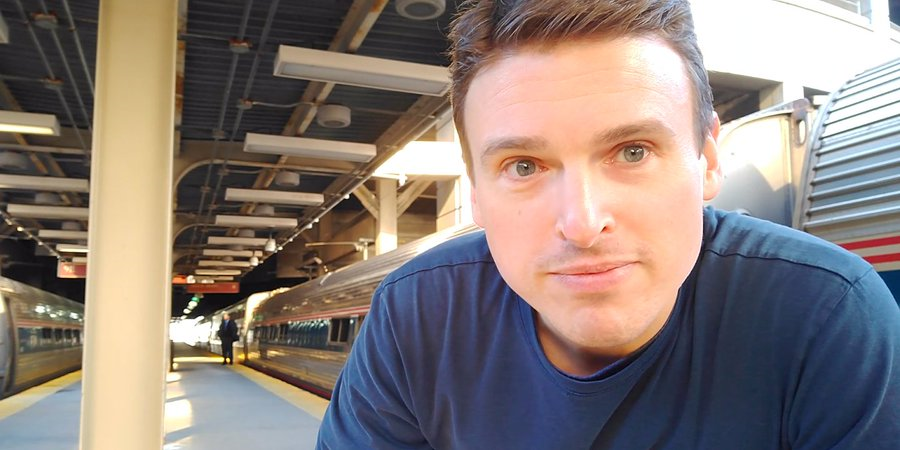
A California scientist studies Earth’s atmosphere in search of clues to modeling a warming world
A shallow cumulus cloud, the kind you might see in a child’s drawing, is a puffball with a flat bottom and a cauliflower top.
That’s according to David Romps, a professor of Earth and planetary science at the University of California, Berkeley. He then adds context to these common but critical clouds: when considered collectively, shallow cumuli provide significant shade for the Earth by reflecting sunlight back into space. According to one estimate, without such clouds, planetary surface temperatures would be nearly 10 degrees Fahrenheit warmer than they are now.
But does a shallow cumulus cloud behave like a bubble or a plume? That is, does such a cloud act like a soon-dissipated pocket of isolated air or more like a long-lasting fountain of air?
To Romps and many other atmospheric scientists, that is an important question. The fate of air ascending through a cloud’s base determines how big the cloud will get and how long it will last. Size and longevity determine a cloud’s albedo (reflectivity) and, therefore, its climate impact.
The bubble-or-plume answer is complicated.
In a recent paper on the life cycle of shallow cumulus clouds, Romps and others gained some insights by using data from stereo cameras, Doppler lidar, and large-eddy simulations. They concluded that observed clouds tend to behave like short-lived bubbles, but simulated clouds are longer-lived, more active, and plume-like.
Because the models clash with the observations, says Romps, “there is room for improvement” in how such clouds are represented in earth system models (ESM).
Making such improvements is one mission of the Atmospheric System Research (ASR) program at the U.S. Department of Energy (DOE). Accordingly, ASR was first in a list of the paper’s acknowledgments.
Data for the bubble-plume study came from the Southern Great Plains (SGP) atmospheric observatory, which spans parts of Oklahoma and Kansas. It’s the oldest and largest such site run by DOE’s Atmospheric Radiation Measurement (ARM) user facility.
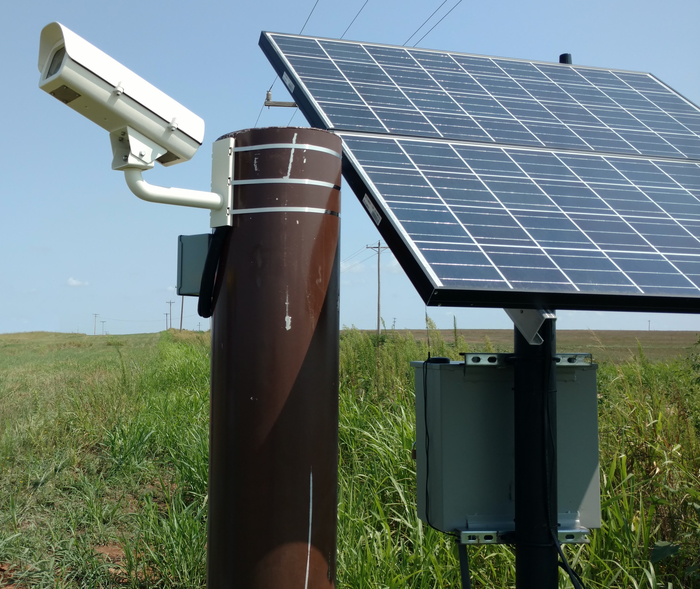
ARM measurements often inform the wide net of research Romps has cast since 2011, the year he arrived at Berkeley. And ASR is often among his funders.
Deepening his DOE connections, Romps also has a joint appointment with the agency-funded Lawrence Berkeley National Laboratory.
Modernized, Digitized Stereophotogrammetry
So far in 2021, Romps has led, or has been part of, 10 papers.
Beyond cloud shape and behavior, he and co-authors have also looked at turbulent plumes, convective aggregation, and the impact of global warming on severe thunderstorms. They have investigated moist thermodynamics, fire risk from arctic lightning, and how a new algorithm can distinguish swarms of insects from clouds of liquid water.
The insect paper, published in February 2021, was led by Christopher R. Williams at the University of Colorado, Boulder. Romps and his frequent collaborator, Berkeley project scientist Rusen Öktem, were among the co-authors. They used stereo cameras they put in place at the SGP to help confirm the algorithm’s efficacy.
Romps and Öktem compared data reports to cloud heights captured by their three-pair STEREOCAM array at the SGP.
In particular, the co-authors used a Clouds Optically Gridded by Stereo (COGS) data product from a Berkeley cloud science team directed by Romps. At the SGP, every 20 seconds, stereo cameras supplying COGS data record a four-dimensional (4D) grid of cloudiness that is 6 kilometers (3.7 miles) to a side, yielding a volume of around 200 cubic kilometers (50 cubic miles).
In a 4D grid, three of the dimensions are spatial; the fourth is time.
Romps revived and modernized a measurement technology called stereophotogrammetry, first used in the nineteenth century. He and Öktem redesigned camera placement and calibration. They also devised an algorithm that can reconstruct passing clouds.
A pair of digital stereocams about 120 degrees apart and set at prescribed angles are used to reconstruct the shape, height, and upward velocity of clouds.
The technology, now widely associated with research by Romps, can calculate the speed at which clouds rise through the atmosphere. (That’s difficult to measure.) The paired cameras can also quantify cloud boundaries without the traditional aid of an orienting landmark.
No need for such a landmark means that the Romps version of stereocam technology can be applied to marine clouds, which inhabit the air above featureless expanses of ocean.
‘The First Inkling’
Romps says “the first inkling” that this could be a useful way to study clouds happened on a beach in Mexico. In 2011, he and his wife were staying at a very expensive resort, “with not much money left for excursions,” says Romps. “But there was beautiful convection there,” visible from his perch on a beach towel. “At arm’s length, I could hold my thumb against the cloud base, watch as the cloud top rose, and roughly estimate the cloud’s vertical velocity.”
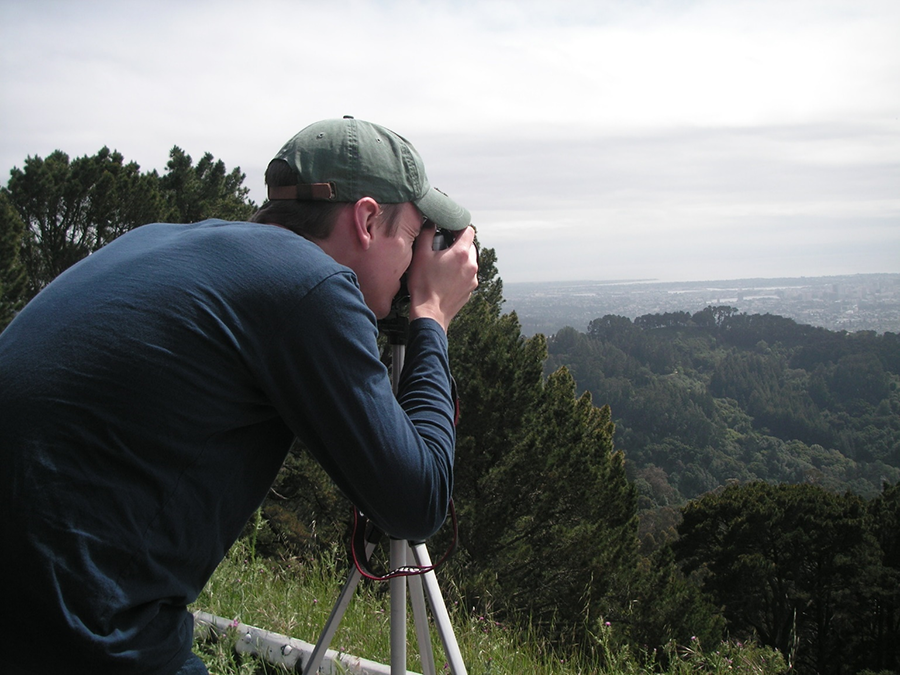
From there, he says, “I started playing around with my own camera” from roadside ocean lookouts between Berkeley and Oakland, California. By 2014, Romps and Öktem published a paper introducing a zero-landmark calibration technique that extended ground-based stereophotogrammetry to oceanic settings.
The 2013 experiment described in the study used two off-the-shelf security cameras placed about 1 kilometer (0.6 miles) apart on Biscayne Bay in Miami, Florida. The pair created stereo images that enabled Romps to reconstruct cloud-base heights, which were then verified by lidar and radiosondes.
At the SGP, six stereo cameras arrayed in a ring use the same low-tech, low-cost digital camera systems. Each weather-shielded camera, the size of a toaster, is mounted on a sturdy post, powered by a solar panel, and hooked to a battery, computer, and a GPS.
Co-aimed at objects as far as 30 kilometers (18.6 miles) away, one paired camera system can capture spatial resolution images as fine as 50 meters (164 feet) across. In atmospheric science terms, that is fine-grained data.
Since the camera systems are automated, autonomous, inexpensive, and small, “you can put them about anywhere,” says Romps, who envisions the technology becoming a staple of atmospheric observatories and campaigns everywhere.
He is ARM’s lead mentor for the cameras; Öktem is co-mentor.
When it comes to participating in field campaigns, says Romps, “we are ready to leap into action.”
In Argentina, a First Outing
For now, ARM has two other stereo cameras in its arsenal, deployable as a pair as part of the 50-instrument first ARM Mobile Facility (AMF1). The cameras were active during ARM’s 2018–2019 Cloud, Aerosol, and Complex Terrain Interactions (CACTI) field campaign in Argentina.
“It was a really nice testbed,” says Romps, whose stereo cameras helped retrieve data on the heights of cloud boundaries.
Rendered into charts, the data showed vertically rising columns of air that were like “turrets on a castle,” he says, which typically mark a cloud’s highest-reaching element.
Romps and Öktem were among the co-authors of a 2021 paper on convective cloud transitions during CACTI, which included stereophotogrammetry data from camera sites deployed within 2 kilometers (1.2 miles) of AMF1.
The same two cameras will soon be part of the TRacking Aerosol Convection interactions ExpeRiment (TRACER). The ARM field campaign will launch in October 2021 in Houston, Texas, where seasonal storms are frequent and dramatic.
“We’ll get an unobstructed view of the main site,” says Romps of the ASR-funded TRACER work, and that view will yield “a bigger picture of cloud fractions and sizes as storms grow and dissipate.”
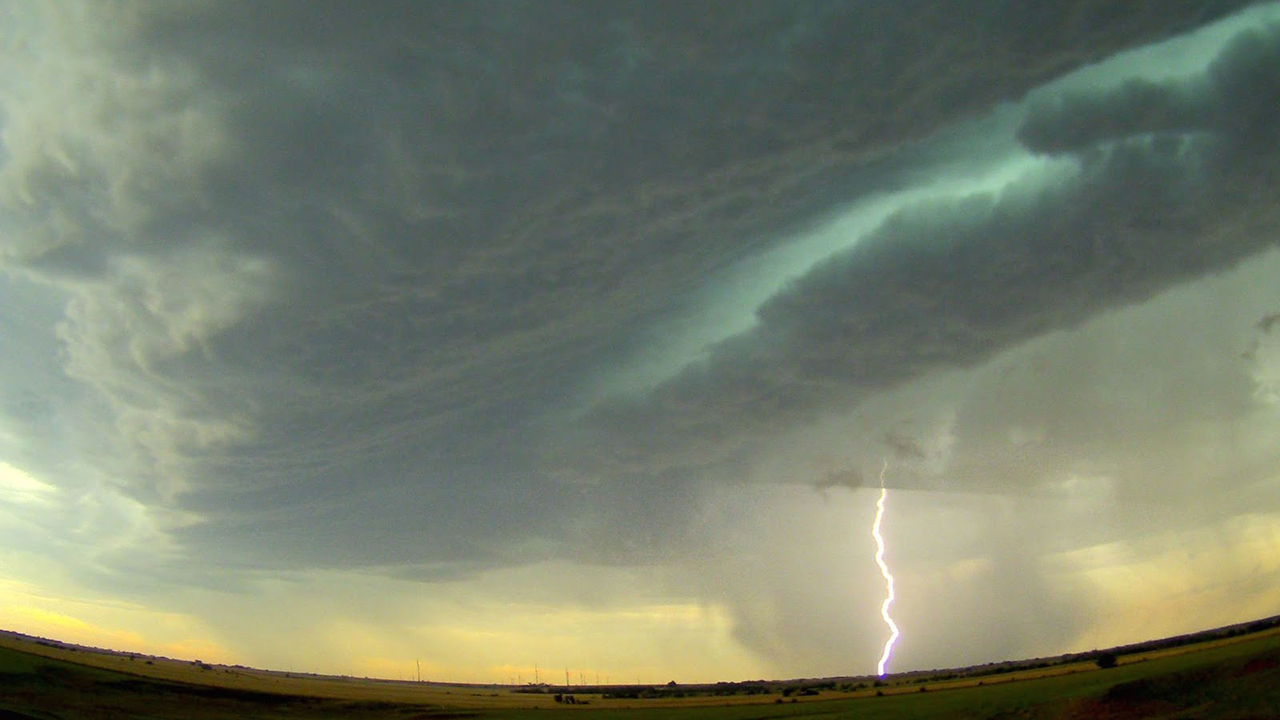
Satellites were paired with stereo cameras in another 2021 paper, this one on shallow cumulus cloud detection at the SGP, co-authored by both Romps and Öktem. They used COGS data to validate the results of a NASA satellite system’s theoretical ability to see such clouds.
The GOES-16 weather satellite turned out to be good at it, which opens up what Romps says is a new datastream for studying cloud dynamics.
Lightning and CAPE
From 2014 to 2021, Romps led a series of four interconnected papers (and co-authored a fifth) on the predicted increase in the energy available to storms on a warming planet. The consequences of such energy increases include more wind, hail, and lightning.
A 2014 paper Romps led, published in Science, projected an increase in lightning strikes over the continental United States because of global warming. The authors proposed a formula for predicting the rate of such a rise: the convective available potential energy (CAPE) times the rate of precipitation (CAPE x P).
CAPE is a measure of potential storm energy and an indicator of “how explosively clouds can rise,” wrote Romps in a summary of the paper. Taken another way: Imagine CAPE as the measure of the buoyancy of a parcel of air that rises from near the surface of the Earth through the troposphere.
A 2015 paper co-authored by Romps and his one-time PhD student Jacob Seeley takes on the question of why CAPE increases with temperature.
They found an unexpected reason: increased buoyancy in the upper troposphere, where the tropopause undergoes a rapid lifting linked to surface temperatures. (The tropopause marks the outer limit of the troposphere, which borders the stratosphere.)
In 2016, Romps was the sole author on a paper that laid out the first analytical solution to a moist convective atmosphere of the kind that makes increases in CAPE possible. Surprisingly, the effect of warming on CAPE has a limit, he discovered. The limit is 310K (about 98 degrees Fahrenheit) and gets even lower as temperatures rise after that.
Arctic Risks
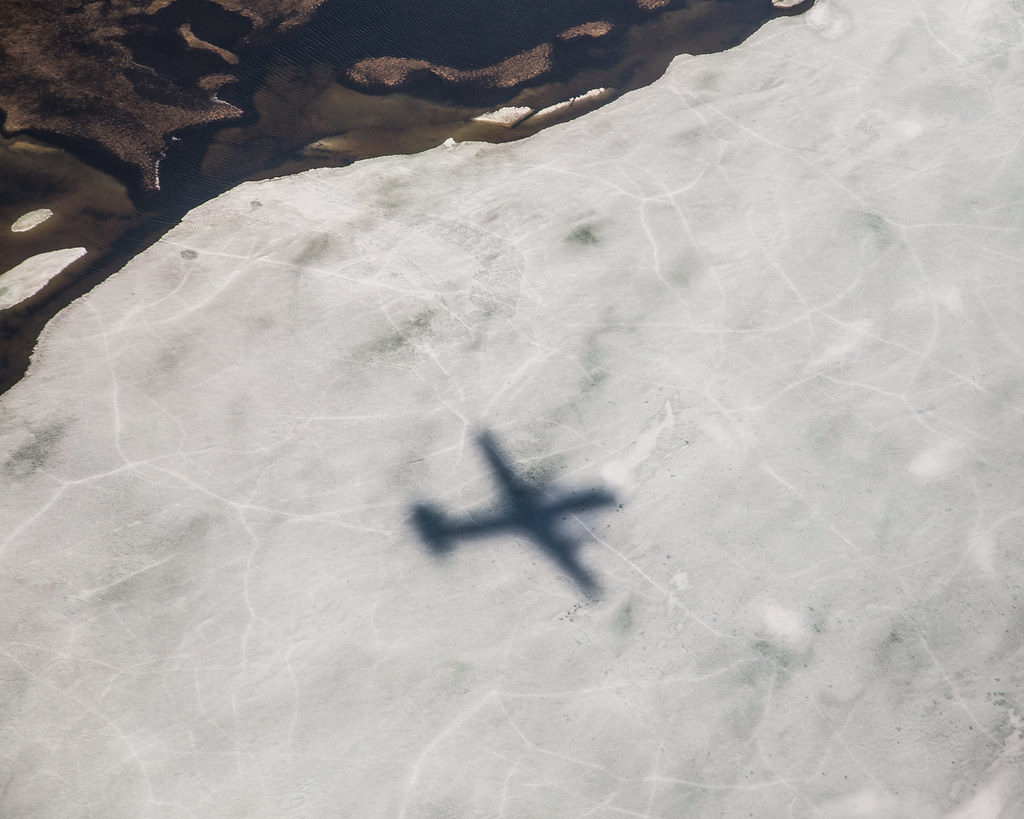
The last two papers in what might be called the CAPE series were struck by lightning.
In a solo paper from 2019, Romps focused his inquiries on the “future of lightning” in models that resolve (represent) clouds. He compared his simulated results to outputs from ESM, in part by using two proxies for the rate of lightning strikes.
One proxy was his own CAPE x precipitation (CAPE x P) formula, which predicts a significant increase in lightning over the continental United States and tropical oceans. The other proxy is based on ice lofted into the atmosphere.
When used in cloud-resolving simulations instead of ESM, both proxies predicted large increases in continental lightning.
In 2021, Romps employed a mix of satellite and historical data as co-author of a Nature Climate Change paper that used the CAPE x P proxy to predict a strong increase in summertime lightning rates over northern circumpolar regions.
How strong? As much as 112% more by the end of the century.
“We’re concerned about large increases” in arctic lightning strikes, says Romps.
When paired with an abundance of exposed tundra, more lightning flashing down into arctic regions will likely cause a fire-vegetation feedback that releases more carbon from permafrost soil. In turn, that release of carbon will accelerate planetary warming.
Romps also has wider concerns about a warming Earth―especially an Earth that, as he writes in a 2020 paper, appears to change in abrupt bumps instead of by steady increments.
Two Main Worries
How did Romps get from a doctoral dissertation on the physics of string theory at Harvard (PhD, 2005) to a career in the physics of the climate?
“I decided to work on the climate problem―the most urgent problem of our time.” – David Romps
“My path to what I am doing now was circuitous,” he admits. “In the latter part of my graduate years, I decided to work on the climate problem―the most urgent problem of our time.”
As if to answer that concern, the American Meteorological Society published its annual State of the Climate report in late August 2021. The authors noted a widespread rise in average temperatures across the globe in 2020 and a rise in atmospheric carbon dioxide (CO2) levels, which are at the highest levels in at least the last 800,000 years.
Rising temperatures and CO2 levels are two main worries for Romps. He has pursued research looking into them, including his single-author 2020 paper on reducing the computational cost of some cloud-related greenhouse gas experiments. It touches on a value called equilibrium climate sensitivity (ECS), which (to a point) dramatically increases with higher surface temperatures.
ECS is not infinite, he says in a video explanation of the paper, and “we cannot push Earth to a runaway state by burning fossil fuels. (But) it doesn’t take a full runaway to ruin your day.”
Venus has undergone such a runaway state, he explains, because it was unable to shed increasing levels of heat. Today, its planetary surface is hot enough to melt lead.
‘We Find Ourselves Here’
At Harvard, Romps started researching string theory under the guidance of physicist Andrew Strominger, attracted by a theory “that has the potential to unify the various forces and to explain why the world is the way it is.”
String theory deals with physics in 10 or 11 dimensions, many of which are curled into “loops so small that they require high energies to probe them,” says Romps.
Instead, he turned his focus on what he calls “our very low-energy world. We live in a 4D world. We find ourselves where we are possible. We find ourselves here.”
Romps wrote his dissertation on holography and other topics in string theory, then did a year of postdoctoral research at the Woods Hole Research Center in Massachusetts (2005–2006), where he delved into environmental policy with John Holdren at the Harvard Kennedy School.
It was through Holdren that Romps started met Harvard environmental scientist Daniel Schrag, who steered the young physics PhD into applying his training to the atmosphere.
“He was right,” says Romps.
He then spent a second postdoc at Harvard’s Center for the Environment (2006–2008), where he worked with atmospheric scientist Zhiming Kuang. At the same time, Romps ground his way through a self-imposed crash course on the science of clouds, aerosols, climate simulations, and solar radiation.
A Career Shift
“During my postdoc, I crammed a typical graduate school experience into a very compressed amount of time,” says Romps, who sees his story as a cautionary tale. “In the beginning (while reading the literature), I would highlight the whole paper. It’s not a path I recommend.”
Today, informed by his own experience, Romps is an enthusiastic mentor to graduate students, especially those studying physics.
“I am happy to help them make that transition” to climate science, says Romps, who teaches courses on climate change and atmospheric dynamics. “I get inquiries every year.”
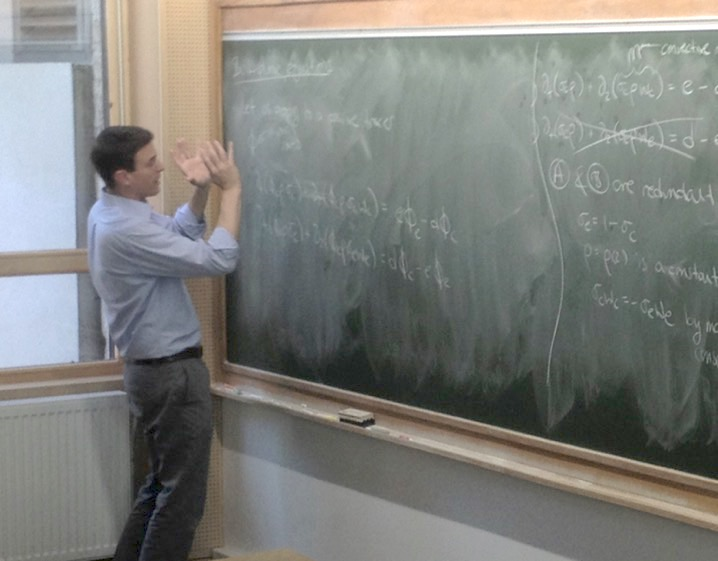
The same spirit of outreach is international, as when he delivered a summer 2017 lecture on tropical moist convection at the Les Houches School of Physics in France, which sponsors such events for early-career scientists. (The Romps lecture notes were published in 2020.)
Waking to the Natural World
Romps penned his first atmospheric science paper in 2008 on the dry-entropy budget of a moist atmosphere. That was the same year he started as a research associate at Harvard (2008 to 2010). Next came Berkeley, where he ascended through the academic ranks to become a full professor in 2018.
Some might argue that Romps started on his path to science at Yale (B.S./M.S. physics; B.S. mathematics, 1999).
“It was the place for me and my passion for physics,” he says.
But the real answer probably lies in various patches of woods in and around west Philadelphia, which influenced a very young Romps.
“Philadelphia was a far cry from a farm, but I was very interested in science and had a deep love for the natural world,” he says.
Romps recalled hikes and fossil hunting and summers in a day camp nestled in one of those patches of woods. “To my parents’ great credit, they fostered my interest in science and nature. (We were) a family that cared about the natural world.”
Waking to a Warming World
His father worked as a clinical psychologist and his mother as a preschool teacher. Both also understood their son’s rapt attention to NASA scientist James Hansen’s 1988 testimony before Congress. (His predictions about global warming have held up more than three decades later.)
“That was a signature moment,” says Romps, now the father of a 10-year-old son. “The world was put on notice that we had actually warmed the planet. At the time, many scientists thought he was going out on a limb.”
Lucky for the world, Romps is digging into the same science―and living it too. A follower on Twitter of #flyingless, he has given up air travel in favor of rail to reduce his personal carbon footprint. (He also runs, bikes and hikes―no fossil fuels required.)
To get where he is, Romps had to cope with a youthful crisis, when “my interest in science was almost snuffed out” by a teacher who could not explain magnets.
He remembers asking, at age 5: “Where are the people who understand how the world works?”
Today, Romps is one of them.
# # #Author: Corydon Ireland, Staff Writer, Pacific Northwest National Laboratory
This work was supported by the U.S. Department of Energy’s Office of Science, through the Biological and Environmental Research program as part of the Atmospheric System Research program.