In his second decade of research, a veteran Colorado researcher has seacoast and arctic cloud studies underway
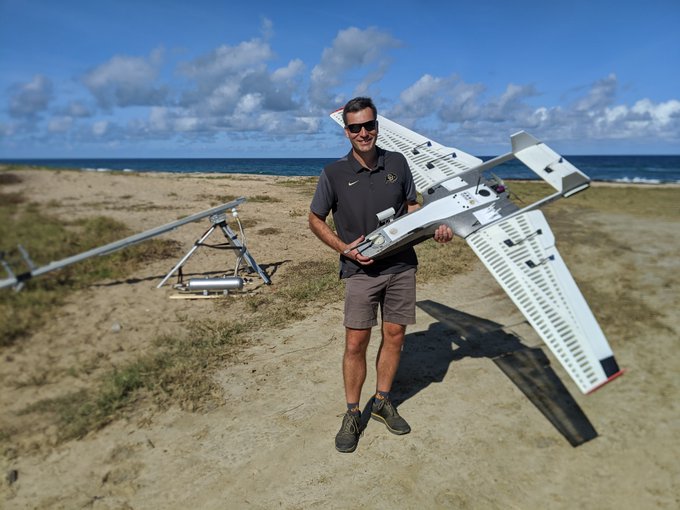
Gijs de Boer is an atmospheric scientist at the University of Colorado, Boulder (CU-Boulder). Much of his research investigates the microphysics of both clouds and the boundary layer they inhabit―inquiries that often involve arctic weather and climate. Fittingly, his first name rhymes with “ice.”
De Boer is 6 feet 4 inches tall, a height he attained by eighth grade. Still, he is not tall enough to get his head into the clouds. For more than a decade he has often gotten there vicariously by mounting instruments on remotely piloted uncrewed aerial systems (UAS).
“We have limited insight into some very critical and key processes,” he says, including the vertical structure of the atmosphere, aerosol processes, and cloud microphysics. “Different processes that drive the life cycle of clouds were under-observed. How do we solve that problem? Flying robots seems like a good idea.”
De Boer does not describe himself as a UAS pioneer since more than a decade ago “I stepped into a world where that was already a thing,” he says.
Yet many in the research world think “de Boer” at the same time they think “UAS,” recalling his wing-and-rotor research not only in the Arctic but also the Caribbean, Oklahoma, and rural Texas.
His vision for a tiny air force of UAS helped de Boer earn a Presidential Early Career Award (PECASE) in 2016, American science’s most prestigious national award for those just starting independent research.
The nimble battery-powered craft in his present-day aerial toolbox includes fixed-wing platforms launched from catapults, such as the RAAVEN platform developed at CU-Boulder. Others are quadcopters that buzz like bees as they yaw, pitch, and accelerate during their sky-sweeping roles as mobile atmospheric sensors.
In August 2023, de Boer will attend the 8th annual meeting of the International Society for Atmospheric Research using Remotely piloted Aircraft (ISARRA) in Norway. (He is on the 2023 ISARRA scientific board.)
Aerial-Aided ASR Work
UAS are at the heart of a 2020-2023 project de Boer has underway on behalf of the Atmospheric System Research (ASR) program at the U.S. Department of Energy (DOE).
“Different processes that drive the life cycle of clouds were under-observed. How do we solve that problem? Flying robots seems like a good idea.”
– Gijs de Boer
The object is to learn more about atmospheric properties at work just above the Earth’s surface in an urban-rural region where breezes from a nearby ocean influence the convective clouds that generate storms.
According to NASA, about a third of the people on Earth live within 60 miles of an ocean coastline. Convective clouds help determine how much energy reaches the Earth’s surface, deliver rain, and distribute surface heat and energy.
Representing them accurately in earth system models is important, though difficult in a warming world riven with complex aerosol heterogeneities. Improved models are central to the ASR funding mission.
Slow-flying, low-flying, low-cost UAS add valuable measurement capabilities. They complement cloud-evolution data from satellites, piloted aircraft, tethered balloons, and ground-based instruments.
De Boer says his involvement with UAS “benefited from the wave of technological advancement in miniaturization” just getting underway in the late 2000s when he began his career. Cell phones were getting smaller and advanced batteries were nudging gas-powered UAS to the side.
Joining TRACER
The UAS de Boer employs in his ASR project fly in computer-controlled aerial transects at different altitudes within the first half-mile or so of the boundary layer. Miniaturized instruments record data on temperature, winds, humidity, and aerosols. (Aerosols are tiny atmospheric particles that influence cloud formation, precipitation, and storms.)
Of course, collecting atmospheric data requires fieldwork. De Boer signed on as a co-investigator with the one-year, all-season Tracking Aerosol Convection Interactions Experiment (TRACER), a 2021―2022 field campaign led by Mike Jensen at Brookhaven National Laboratory.
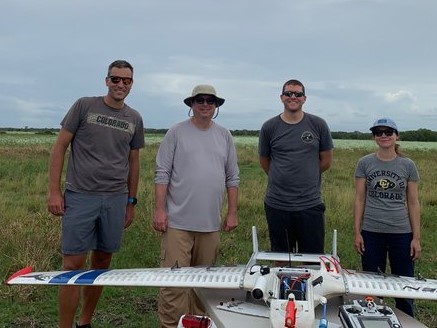
Its core instrumentation, supplemented by guest instruments from collaborators, was supplied by Atmospheric Radiation Measurement (ARM), a DOE user facility with fixed-location and mobile observatories across the world.
TRACER is now in its data-analysis phase, but in the field was a sprawling and comprehensive presence in the urban-rural-coastal Houston, Texas, region. There were 32 co-principal investigators during a year of multi-institution collaboration. More than a dozen satellite campaigns, many supported by ASR, added complementary perspectives and instruments.
One of those sister campaigns was de Boer’s project to capture a picture of land and sea breeze interactions between Houston itself and the Gulf of Mexico. In this region of pre-convective activity, his team took their measurements during TRACER’s four-month summer 2022 intensive operations period (IOP). Among other things, the area’s summer guarantees an interval of historically heightened convective activity.
Mechanisms of Convection
During the TRACER IOP, de Boer and his team of UAS aeronauts launched UAS on transects over private property adjacent to the Brazoria National Wildlife Refuge. They deployed two main aircraft. One was the four-rotor CopterSonde, developed at the University of Oklahoma. Weighing in at about 5 pounds, it can fly for about 18 minutes at a time.
A RAAVEN, with its 91-inch wingspan, can cruise for about 2.5 hours at 38 mph, though its top speed is twice as fast. It was especially useful for getting high-frequency measurements of turbulence.
“It was also one of the few sources of information on the vertical structure of aerosol properties,” says de Boer of his UAS. “There weren’t many sensors (at TRACER) regularly sampling the lower atmosphere in that way.”
With data in hand now from routine flights at about 600 meters above the ground, de Boer’s team is pursuing many science questions. Among them: Before convective clouds form, how do the properties of the boundary layer influence cloud formation and storm intensity?
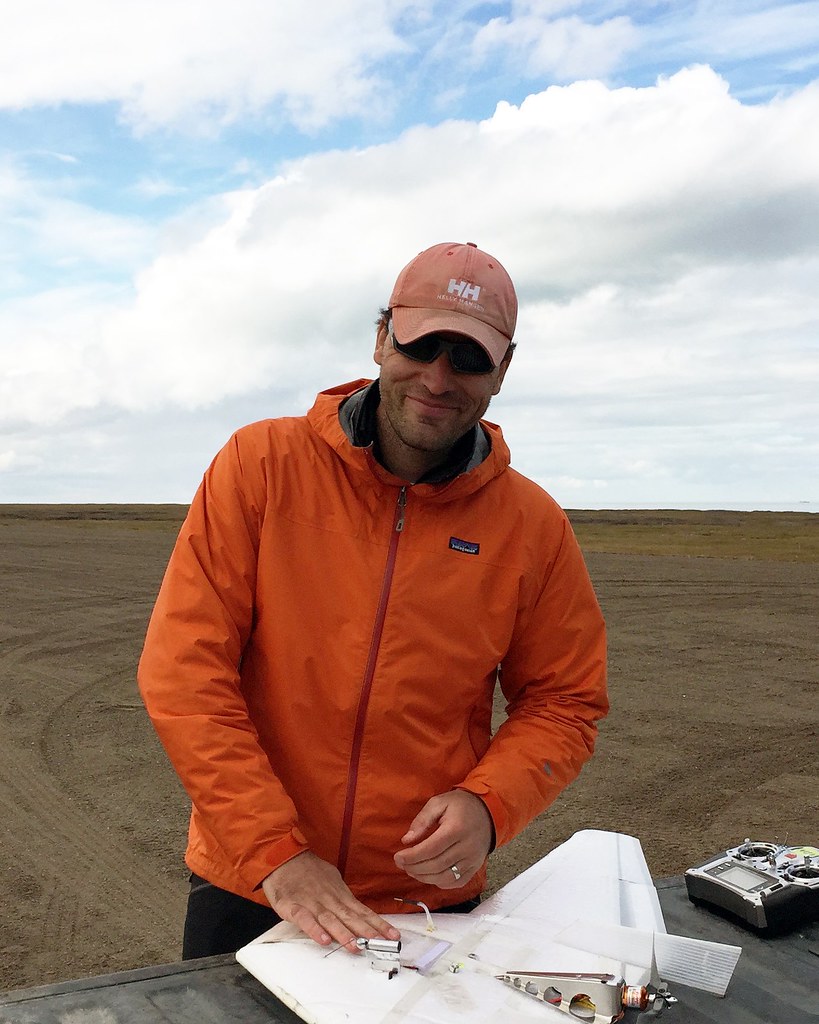
“We were interested in the mechanisms responsible for generating convection in this coastal regime,” he says.
Of particular interest is the interface between the continental boundary layer and the sea-breeze frontal zone, which has enhanced updrafts and turbulence.
A Cold-Weather Start
Texas can be hot and breezy, but de Boer first used UAS for atmospheric research in one of the most hostile environments for such flying imaginable: windy, frigid Oliktok Point on the North Slope of Alaska. An ARM mobile observatory operated there for eight years until the fall of 2021.
“That was my first exposure” to UAS for research, says de Boer.
He led a 2015―2016 ARM field campaign called Evaluation of Routine Atmospheric Sounding Measurements using Unmanned Systems (ERASMUS). It marked his abiding interest in how little was still known about mixed-phase clouds in the Arctic, the subject of his doctoral dissertation. ERASMUS was also a natural laboratory for UAS, an adventure de Boer has recorded in vivid, picture-rich blogs.
Again, no pioneering. Atmospheric scientists had used UAS for “non-arctic purposes,” he says, at least 20 years before ERASMUS, including ARM, which first flew such aircraft in 1991.
During a two-week ERASMUS interval in 2015, de Boer and his team deployed the DataHawk (later the DataHawk2), a lightweight, inexpensive aircraft with a wingspan of about 3 feet. It swept up data on temperature, humidity, pressure, and wind speeds in the lower arctic atmosphere, including when sea ice began to form.
In 2016, the ERASMUS team used a larger, heavier Pilatus UAS for the same kinds of data, as well as measures of radiative and aerosol conditions during a period of arctic haze.
In all, says de Boer, ERASMUS “helped shape ARM’s current aerial capabilities” during what was “a trial run of smaller and cheaper platforms” than larger UAS flying today.
However, in de Boer’s conception of UAS utility small remains beautiful―at ARM and elsewhere.
“There is still a lot of excitement about these kinds of smaller UAS platforms,” he says.
From Cold to Complex
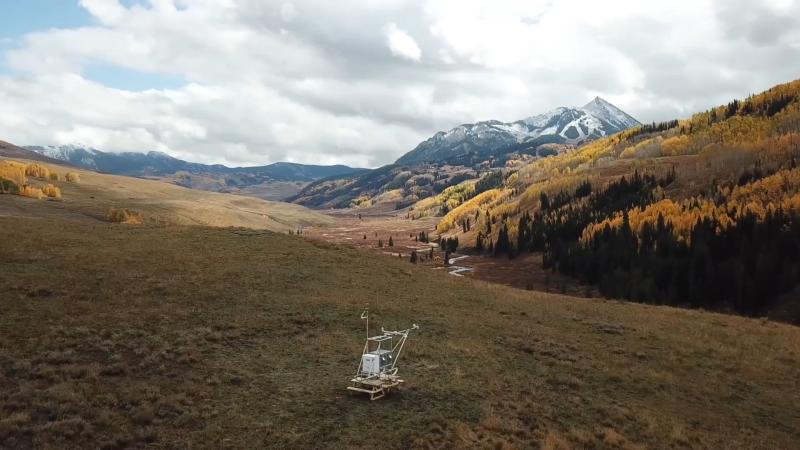
De Boer kept up the UAS drumbeat by taking part in a series of field experiments that included arctic investigations and, eventually, complex mountain terrain.
In 2016, at Oliktok Point, the Inaugural Campaigns for ARM Research using Unmanned Systems (ICARUS) combined UAS and surface instruments with tethered balloon systems (TBS) to capture big-picture data on the fast-changing arctic environment.
In 2018, de Boer led an ARM campaign called Profiling at Oliktok Point to Enhance YOPP Experiments (POPEYE). It deployed UAS, TBS, and surface instruments during a period of focused observation and modeling for the Year of Polar Prediction (YOPP).
A team de Boer led was also part of the 2019―2020 Multidisciplinary Drifting Observatory for the Study of Arctic Climate (MOSAiC), an epically comprehensive, 500-scientist international investigation of shrinking ice and other conditions in the Central Arctic. The DOE, including ASR, was an early MOSAiC supporter.
DataHawk aircraft from CU-Boulder collected fine-grained atmospheric data on wind velocities, turbulence, temperature, humidity, and other measures connected to rapid climate change in the Arctic. Simultaneously, a HELiX multicopter mapped out spatial differences and gradients of the ice surface to help understand how much energy from the sun is reflected back to space.
In the fall of 2021, de Boer threw the switch on a five-site network of air-surface-subsurface instrumentation for a National Oceanic and Atmospheric Administration (NOAA)-funded field campaign in Colorado’s East River Watershed called the Study of Precipitation, the Lower Atmosphere and Surface for Hydrometeorology (SPLASH). Arranged to capture different scales of variability, from 300 meters to several kilometers, SPLASH instruments will run through the fall of 2023.
Data on rain, snow, clouds, soil moisture, and other factors will improve weather and water-runoff predictions in a region facing water shortfalls in a warming climate.
Among the SPLASH panoply of observation technologies are UAS, including RAAVEN, the rotary-wing HELiX deployed at MOSAiC, and a slim, silvery fixed-wing UAS from Black Swift Technologies. In the spring of 2022, flights measured the evolution of surface albedo during the season’s historical melt regime. That summer, radiometer-equipped Black Swift flights took in data on soil moisture.
“We’ve been focused on surface characterization,” says de Boer of UAS during SPLASH, while other instruments look closely at the atmosphere.
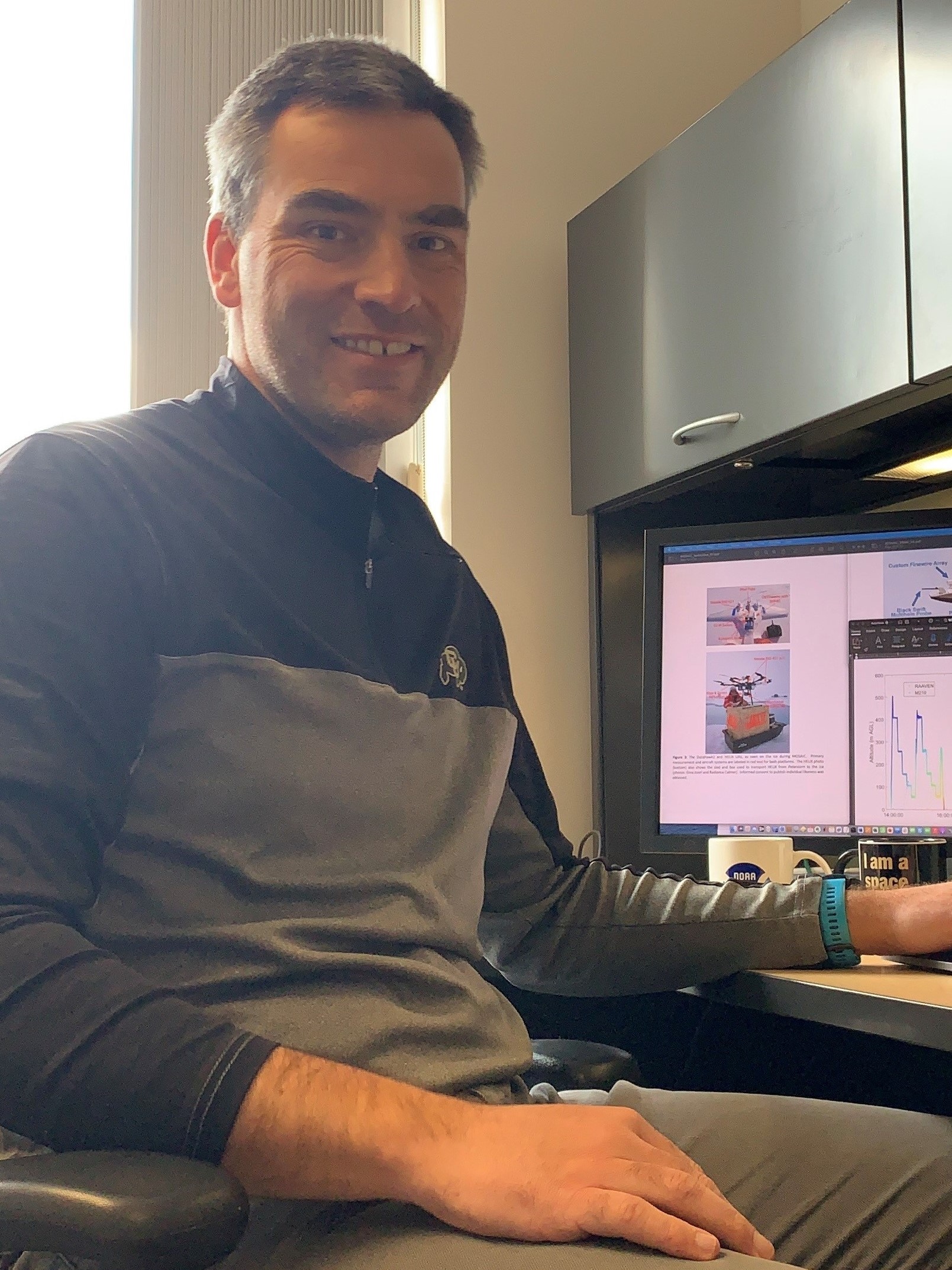
SPLASH is co-located with ARM’s Surface Atmosphere Integrated Field Laboratory (SAIL), an ASR-supported running at the same time with a complementary science mission.
SPLASH and SAIL’s Colorado site, he says, “is right in our backyard” and will create enduring collaborations beyond 2023.
Watching the Weather
De Boer was born in the Netherlands, where his father roved the world as a sea captain with the Dutch merchant marine. It was one influence, he says, that kept him interested in the weather at an early age. Another, eventually, was wanting to know when his soccer games might get rained out.
The family moved to Connecticut when de Boer was five years old.
At Guilford High School, he played soccer on a very good team. “I was proud to play occasionally,” he says, but basketball “was probably my better talent.”
At Cornell University (B.S. atmospheric science, 2002), de Boer set out to be a television weatherman, rounding out his studies by rowing for the university’s varsity heavyweight eight. Then, in the summer of 2001, right before starting his senior year, de Boer did an internship at Cornell’s Northeast Regional Climate Center, where he says he “may have been one of the first ever to program in Python.”
A few months later, he applied to several graduate schools to go beyond television and to test an inclination towards oceanography.
At the University of Wisconsin-Madison (atmospheric science M.S., 2004, PhD, 2009), de Boer was drawn not to the ocean but to lake breezes, particularly those off Lake Michigan in the wintertime.
His mentors, modeler Gregory Tripoli and lidar expert Edwin Eloranta, were involved in the Lake-Induced Convection Experiment (Lake-ICE) looking coastal circulations and the evolution of convection over Lake Michigan.
“That was exciting to me,” says de Boer, especially as a bridge between modeling and observations.
Tripoli had developed a nonhydrostatic model of lake-induced circulations that influenced de Boer’s master’s thesis. Eloranta, meanwhile, had just sent one of his lidar systems to ARM’s North Slope of Alaska atmospheric observatory.
“I got my connection to both the Arctic as well as ARM at that point,” says de Boer, who went on to write a dissertation based on data from a landmark high-latitudes ARM field campaign called the Mixed-Phase Arctic Cloud Experiment (M-PACE).
M-PACE included a sister campaign led by Eloranta that used high spectral resolution lidar the University of Wisconsin-Madison lidar team had developed to measure cloud optical depth and backscatter. In turn, Eloranta’ s campaign informed a string of papers de Boer coauthored through 2011, including one in 2008 on the state of sensor technology used to measure stratiform mixed-phase clouds.
This turn towards arctic clouds, says de Boer, “kind of kick-started the next 10 years or so.”
This stuff that we do is super-important. It helps us to predict what is going to happen five minutes from now, what’s going to happen tomorrow, and potentially to predict what’s going to happen 100 years from now.”
– Gijs de Boer
‘This Stuff That We Do’
Those M-PACE papers from 2008 to 2011 included coauthor Matthew Shupe, a fast-rising arctic researcher at CU-Boulder who was part of de Boer’s pathway to the same university.
Right after getting his PhD, however, de Boer spent two years at Lawrence Berkeley National Laboratory (LBNL), which he says “helped me better understand the national lab environment and how (such labs) operate.”
He worked on arctic processes with climate scientist Surabi Menon until she left for the non-profit ClimateWorks Foundation in San Francisco. Then de Boer joined projects led by LBNL climate modeler William Collins, including investigations of aerosol-cloud interactions in the Arctic.
In 2011, de Boer, his wife (a landscape architect), and his infant daughter moved to Boulder. (He has two children now, ages 11 and 9, and he coaches his daughter’s basketball team.)
His office is two doors away from Shupe’s. Both share an affiliation with NOAA’s Physical Sciences Laboratory in the Cooperative Institute for Research in Environmental Sciences (CIRES), a partnership between CU-Boulder and NOAA.
At the university, de Boer is associate director for science at the Integrated Remote and In-Situ Sensing (IRISS) initiative, which is primarily focused on UAS and supplied the different types of uncrewed aircraft used during MOSAiC. He is also director of National Laboratory Partnerships at CU-Boulder’s Research and Innovation Office, designed to foster research collaborations with the broader DOE landscape.
“This stuff that we do is super-important,” says de Boer, looking back at his decade-plus of observations and modeling. “It helps us to predict what is going to happen five minutes from now, what’s going to happen tomorrow, and potentially to predict what’s going to happen 100 years from now.”
# # #Author: Corydon Ireland, Staff Writer, Pacific Northwest National Laboratory
This work was supported by the U.S. Department of Energy’s Office of Science, through the Biological and Environmental Research program as part of the Atmospheric System Research program.